List_of_fusion_experiments
List of fusion experiments
List of efforts toward artificial nuclear fusion
Experiments directed toward developing fusion power are invariably done with dedicated machines which can be classified according to the principles they use to confine the plasma fuel and keep it hot.
![]() | This article has multiple issues. Please help improve it or discuss these issues on the talk page. (Learn how and when to remove these template messages)
|

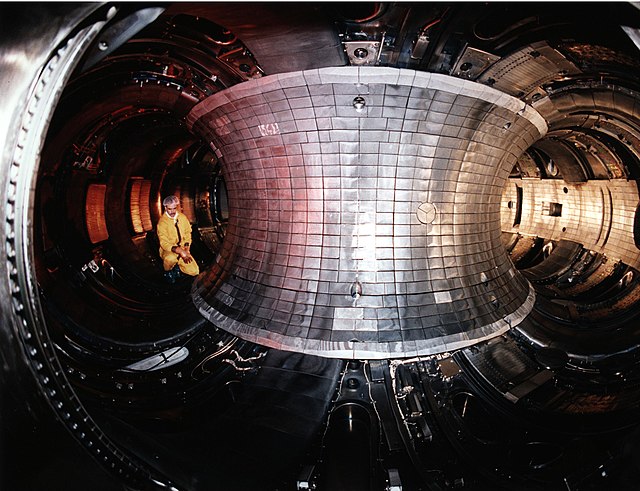
The major division is between magnetic confinement and inertial confinement. In magnetic confinement, the tendency of the hot plasma to expand is counteracted by the Lorentz force between currents in the plasma and magnetic fields produced by external coils. The particle densities tend to be in the range of 1018 to 1022 m−3 and the linear dimensions in the range of 0.1 to 10 m. The particle and energy confinement times may range from under a millisecond to over a second, but the configuration itself is often maintained through input of particles, energy, and current for times that are hundreds or thousands of times longer. Some concepts are capable of maintaining a plasma indefinitely.
In contrast, with inertial confinement, there is nothing to counteract the expansion of the plasma. The confinement time is simply the time it takes the plasma pressure to overcome the inertia of the particles, hence the name. The densities tend to be in the range of 1031 to 1033 m−3 and the plasma radius in the range of 1 to 100 micrometers. These conditions are obtained by irradiating a millimeter-sized solid pellet with a nanosecond laser or ion pulse. The outer layer of the pellet is ablated, providing a reaction force that compresses the central 10% of the fuel by a factor of 10 or 20 to 103 or 104 times solid density. These microplasmas disperse in a time measured in nanoseconds. For a fusion power reactor, a repetition rate of several per second will be needed.
Within the field of magnetic confinement experiments, there is a basic division between toroidal and open magnetic field topologies. Generally speaking, it is easier to contain a plasma in the direction perpendicular to the field than parallel to it. Parallel confinement can be solved either by bending the field lines back on themselves into circles or, more commonly, toroidal surfaces, or by constricting the bundle of field lines at both ends, which causes some of the particles to be reflected by the mirror effect. The toroidal geometries can be further subdivided according to whether the machine itself has a toroidal geometry, i.e., a solid core through the center of the plasma. The alternative is to dispense with a solid core and rely on currents in the plasma to produce the toroidal field.
Mirror machines have advantages in a simpler geometry and a better potential for direct conversion of particle energy to electricity. They generally require higher magnetic fields than toroidal machines, but the biggest problem has turned out to be confinement. For good confinement there must be more particles moving perpendicular to the field than there are moving parallel to the field. Such a non-Maxwellian velocity distribution is, however, very difficult to maintain and energetically costly.
The mirrors' advantage of simple machine geometry is maintained in machines which produce compact toroids, but there are potential disadvantages for stability in not having a central conductor and there is generally less possibility to control (and thereby optimize) the magnetic geometry. Compact toroid concepts are generally less well developed than those of toroidal machines. While this does not necessarily mean that they cannot work better than mainstream concepts, the uncertainty involved is much greater.
Somewhat in a class by itself is the Z-pinch, which has circular field lines. This was one of the first concepts tried, but it did not prove very successful. Furthermore, there was never a convincing concept for turning the pulsed machine requiring electrodes into a practical reactor.
The dense plasma focus is a controversial and "non-mainstream" device that relies on currents in the plasma to produce a toroid. It is a pulsed device that depends on a plasma that is not in equilibrium and has the potential for direct conversion of particle energy to electricity. Experiments are ongoing to test relatively new theories to determine if the device has a future.
Toroidal machine
Toroidal machines can be axially symmetric, like the tokamak and the reversed field pinch (RFP), or asymmetric, like the stellarator. The additional degree of freedom gained by giving up toroidal symmetry might ultimately be usable to produce better confinement, but the cost is complexity in the engineering, the theory, and the experimental diagnostics. Stellarators typically have a periodicity, e.g. a fivefold rotational symmetry. The RFP, despite some theoretical advantages such as a low magnetic field at the coils, has not proven very successful.
Tokamak[1]
Device name | Status | Construction | Operation | Location | Organisation | Major/minor radius | B-field | Plasma current | Purpose | Image |
---|---|---|---|---|---|---|---|---|---|---|
T-1 (Tokamak-1)[2] | Shut down | 1957 | 1958–1959 | ![]() | Kurchatov Institute | 0.625 m/0.13 m | 1 T | 0.04 MA | First tokamak | ![]() |
T-2 (Tokamak-2)[2] | Recycled →FT-1 | 1959 | 1960–1970 | ![]() | Kurchatov Institute | 0.62 m/0.22 m | 1 T | 0.04 MA | ||
T-3 (Tokamak-3)[2] | Shut down | 1960 | 1962–? | ![]() | Kurchatov Institute | 1 m/0.12 m | 3.5 T | 0.15 MA | Overcame Bohm diffusion by a factor of 10, temperature 10 MK, confinement time 10 ms | |
T-5 (Tokamak-5)[2] | Shut down | ? | 1962–1970 | ![]() | Kurchatov Institute | 0.625 m/0.15 m | 1.2 T | 0.06 MA | Investigation of plasma equilibrium in vertical and horizontal direction | |
TM-1 | Shut down | ? | ? | ![]() | Kurchatov Institute | |||||
TM-2 | Shut down | ? | 1965 | ![]() | Kurchatov Institute | |||||
TM-3 | Shut down | ? | 1970 | ![]() | Kurchatov Institute | |||||
FT-1[2] | Recycled →CASTOR | T-2 | 1972–2002 | ![]() | Ioffe Institute | 0.62 m/0.22 m | 1.2 T | 0.05 MA | ||
ST (Symmetric Tokamak) | Shut down | Model C | 1970–1974 | ![]() | Princeton Plasma Physics Laboratory | 1.09 m/0.13 m | 5.0 T | 0.13 MA | First American tokamak, converted from Model C stellarator | |
T-6 (Tokamak-6) | Shut down | ? | 1970–1974 | ![]() | Kurchatov Institute | 0.7 m/0.25 m | 1.5 T | 0.22 MA | ||
TUMAN-2, 2A | Shut down | ? | 1971–1985 | ![]() | Ioffe Institute | 0.4 m/0.08 m | 1.5 T | 0.012 MA | ||
ORMAK (Oak Ridge tokaMAK) | Shut down | 1971–1976 | ![]() | Oak Ridge National Laboratory | 0.8 m/0.23 m | 2.5 T | 0.34 MA | First to achieve 20 MK plasma temperature | ![]() | |
Doublet II | Shut down | 1972–1974 | ![]() | General Atomics | 0.63 m/0.08 m | 0.95 T | 0.21 MA | |||
ATC (Adiabatic Toroidal Compressor) | Shut down | 1971–1972 | 1972–1976 | ![]() | Princeton Plasma Physics Laboratory | 0.88 m/0.11 m | 2 T | 0.05 MA | Demonstrate compressional plasma heating | ![]() |
T-9 (Tokamak-9) | Shut down | ? | 1972–1977 | ![]() | Kurchatov Institute | 0.36 m/0.07 m | 1 T | |||
TO-1 | Shut down | ? | 1972–1978 | ![]() | Kurchatov Institute | 0.6 m/0.13 m | 1.5 T | 0.07 MA | ||
Alcator A (Alto Campo Toro) | Shut down | ? | 1972–1978 | ![]() | Massachusetts Institute of Technology | 0.54 m/0.10 m | 9.0 T | 0.3 MA | ||
JFT-2 (JAERI Fusion Torus 2) | Shut down | ? | 1972–1982 | ![]() | Japan Atomic Energy Research Institute | 0.9 m/0.25 m | 1.8 T | 0.25 MA | ||
Turbulent Tokamak Frascati (TTF, torello) | Shut down | 1973 | ![]() | ENEA | 0.3 m/0.04 m | 1 T | 0.005 MA | Study of turbulent plasma heating | ||
Pulsator[3] | Shut down | 1970–1973 | 1973–1979 | ![]() | Max Planck Institute for Plasma Physics | 0.7 m/0.12 m | 2.7 T | 0.125 MA | Discovery of high-density operation with tokamaks | |
TFR (Tokamak de Fontenay-aux-Roses) | Shut down | 1973–1984 | ![]() | CEA | 0.98 m/0.2 m | 6 T | 0.49 MA | |||
T-4 (Tokamak-4)[2] | Shut down | ? | 1974–1978 | ![]() | Kurchatov Institute | 0.9 m/0.16 m | 5 T | 0.3 MA | Observed fast thermal quench before major plasma disruptions | |
Doublet IIA | Shut down | 1974–1979 | ![]() | General Atomics | 0.66 m/0.15 m | 0.76 T | 0.35 MA | |||
Petula-B | Shut down | ? | 1974–1986 | ![]() | CEA | 0.72 m/0.18 m | 2.7 T | 0.23 MA | ||
T-10 (Tokamak-10)[2] | Operational | 1975– | ![]() | Kurchatov Institute | 1.50 m/0.37 m | 4 T | 0.8 MA | Largest tokamak of its time | ![]() | |
T-11 (Tokamak-11) | Shut down | ? | 1975–1984 | ![]() | Kurchatov Institute | 0.7 m/0.25 m | 1 T | |||
PLT (Princeton Large Torus) | Shut down | 1972–1975 | 1975–1986 | ![]() | Princeton Plasma Physics Laboratory | 1.32 m/0.42 m | 4 T | 0.7 MA | First to achieve 1 MA plasma current | ![]() |
Divertor Injection Tokamak Experiment (DITE) | Shut down | 1975–1989 | ![]() | United Kingdom Atomic Energy Authority | 1.17 m/0.27 m | 2.7 T | 0.26 MA | |||
JIPP T-II | Shut down | ? | 1976 | ![]() | Nagoya University | 0.91 m/0.17 m | 3 T | 0.16 MA | ||
TNT-A | Shut down | ? | 1976 | ![]() | Tokyo University | 0.4 m/0.09 m | 0.42 T | 0.02 MA | ||
T-8 (Tokamak-8)[2] | Shut down | ? | 1976–? | ![]() | Kurchatov Institute | 0.28 m/0.048 m | 0.9 T | 0.024 MA | First D-shaped tokamak | |
Microtor[4] | Shut down | ? | 1976–1983? | ![]() | UCLA | 0.3 m/0.1 m | 2.5 T | 0.12 MA | Plasma impurity control and diagnostic development | |
Macrotor[4] | Shut down | ? | 1970s–80s | ![]() | UCLA | 0.9 m/0.4 m | 0.4 T | 0.1 MA | Understanding plasma rotation driven by radial current | |
TUMAN-3[2] | Operational | ? | 1977– (1990–, 3M) | ![]() | Ioffe Institute | 0.55 m/0.23 m | 3 T | 0.18 MA | Study adiabatic compression, RF and NB heating, H-mode and parametric instability | |
Thor[5] | Shut down | ? | ![]() | University of Milano | 0.52 m/0.195 m | 1 T | 0.055 MA | |||
FT (Frascati Tokamak) | Shut down | 1978 | ![]() | ENEA | 0.83 m/0.20 m | 10 T | 0.8 MA | |||
PDX (Poloidal Divertor Experiment) | Shut down | ? | 1978–1983 | ![]() | Princeton Plasma Physics Laboratory | 1.4 m/0.4 m | 2.4 T | 0.5 MA | ||
ISX-B | Shut down | ? | 1978–1984 | ![]() | Oak Ridge National Laboratory | 0.93 m/0.27 m | 1.8 T | 0.2 MA | Superconducting coils, attempt high-beta operation | |
Doublet III | Shut down | 1978–1985 | ![]() | General Atomics | 1.45 m/0.45 m | 2.6 T | 0.61 MA | |||
T-12 (Tokamak-12) | Shut down | ? | 1978–1985 | ![]() | Kurchatov Institute | 0.36 m/0.08 m | 1 T | 0.03 MA | ||
Alcator C (Alto Campo Toro) | Shut down | ? | 1978–1986 | ![]() | Massachusetts Institute of Technology | 0.64 m/0.16 m | 13 T | 0.8 MA | ||
T-7 (Tokamak-7)[2] | Recycled →HT-7[6] | ? | 1979–1985 | ![]() | Kurchatov Institute | 1.2 m/0.31 m | 3 T | 0.3 MA | First tokamak with superconducting toroidal field coils | |
ASDEX (Axially Symmetric Divertor Experiment)[7] | Recycled →HL-2A | 1973–1980 | 1980–1990 | ![]() | Max-Planck-Institut für Plasmaphysik | 1.65 m/0.4 m | 2.8 T | 0.5 MA | Discovery of the H-mode in 1982 | |
FT-2[2] | Operational | ? | 1980– | ![]() | Ioffe Institute | 0.55 m/0.08 m | 3 T | 0.05 MA | H-mode physics, LH heating | |
TEXTOR (Tokamak Experiment for Technology Oriented Research)[8][9] | Shut down | 1976–1980 | 1981–2013 | ![]() | Forschungszentrum Jülich | 1.75 m/0.47 m | 2.8 T | 0.8 MA | Study plasma-wall interactions | |
TFTR (Tokamak Fusion Test Reactor)[10] | Shut down | 1980–1982 | 1982–1997 | ![]() | Princeton Plasma Physics Laboratory | 2.4 m/0.8 m | 5.9 T | 3 MA | Attempted scientific break-even, reached record fusion power of 10.7 MW and temperature of 510 MK | ![]() |
JFT-2M (JAERI Fusion Torus 2M) | Shut down | ? | 1983–2004 | ![]() | Japan Atomic Energy Research Institute | 1.3 m/0.35 m | 2.2 T | 0.5 MA | ||
JET (Joint European Torus)[11] | Shut down | 1978–1983 | 1983–2023 | ![]() | United Kingdom Atomic Energy Authority | 2.96 m/0.96 m | 4 T | 7 MA | Records for fusion output power 16.1 MW (1997), fusion energy 69 MJ (2023) | ![]() |
Novillo[12][13] | Shut down | NOVA-II | 1983–2004 | ![]() | Instituto Nacional de Investigaciones Nucleares | 0.23 m/0.06 m | 1 T | 0.01 MA | Study plasma-wall interactions | |
JT-60 (Japan Torus-60)[14] | Recycled →JT-60SA | 1985–2010 | ![]() | Japan Atomic Energy Research Institute | 3.4 m/1.0 m | 4 T | 3 MA | High-beta steady-state operation, highest fusion triple product | ||
CCT (Continuous Current Tokamak) | Shut down | ? | 1986–199? | ![]() | UCLA | 1.5 m/0.4 m | 0.2 T | 0.05 MA | H-mode studies | |
DIII-D[15] | Operational | 1986[16] | 1986– | ![]() | General Atomics | 1.67 m/0.67 m | 2.2 T | 3 MA | Tokamak Optimization | ![]() |
STOR-M (Saskatchewan Torus-Modified)[17] | Operational | 1987– | ![]() | Plasma Physics Laboratory (Saskatchewan) | 0.46 m/0.125 m | 1 T | 0.06 MA | Study plasma heating and anomalous transport | ||
T-15[2] | Recycled →T-15MD | 1983–1988 | 1988–1995 | ![]() | Kurchatov Institute | 2.43 m/0.78 m | 3.6 T | 1 MA | First superconducting tokamak, pulse duration 1.5 s | ![]() |
Tore Supra[18] | Recycled →WEST | 1988–2011 | ![]() | Département de Recherches sur la Fusion Contrôlée | 2.25 m/0.7 m | 4.5 T | 2 MA | Large superconducting tokamak with active cooling | ||
ADITYA (tokamak) | Operational | 1989– | ![]() | Institute for Plasma Research | 0.75 m/0.25 m | 1.2 T | 0.25 MA | |||
COMPASS (COMPact ASSembly)[19][20] | Operational | 1980– | 1989– | ![]() | Institute of Plasma Physics AS CR | 0.56 m/0.23 m | 2.1 T | 0.32 MA | Plasma physics studies for ITER | ![]() |
FTU (Frascati Tokamak Upgrade) | Operational | 1990– | ![]() | ENEA | 0.935 m/0.35 m | 8 T | 1.6 MA | |||
START (Small Tight Aspect Ratio Tokamak)[21] | Recycled →Proto-Sphera | 1990–1998 | ![]() | United Kingdom Atomic Energy Authority | 0.3 m/? | 0.5 T | 0.31 MA | First full-sized Spherical Tokamak | ||
ASDEX Upgrade (Axially Symmetric Divertor Experiment) | Operational | 1991– | ![]() | Max-Planck-Institut für Plasmaphysik | 1.65 m/0.5 m | 2.6 T | 1.4 MA | ![]() | ||
Alcator C-Mod (Alto Campo Toro)[22] | Shut down | 1986– | 1991–2016 | ![]() | Massachusetts Institute of Technology | 0.68 m/0.22 m | 8 T | 2 MA | Record plasma pressure 2.05 bar | ![]() |
ISTTOK (Instituto Superior Técnico TOKamak)[23] | Operational | 1992– | ![]() | Instituto de Plasmas e Fusão Nuclear | 0.46 m/0.085 m | 2.8 T | 0.01 MA | |||
TCV (Tokamak à Configuration Variable)[24] | Operational | 1992– | ![]() | École Polytechnique Fédérale de Lausanne | 0.88 m/0.25 m | 1.43 T | 1.2 MA | Confinement studies | ![]() | |
HBT-EP (High Beta Tokamak-Extended Pulse) | Operational | 1993– | ![]() | Columbia University Plasma Physics Laboratory | 0.92 m/0.15 m | 0.35 T | 0.03 MA | High-Beta tokamak | ![]() | |
HT-7 (Hefei Tokamak-7) | Shut down | 1991–1994 (T-7) | 1995–2013 | ![]() | Hefei Institutes of Physical Science | 1.22 m/0.27 m | 2 T | 0.2 MA | China's first superconducting tokamak | |
Pegasus Toroidal Experiment[25] | Operational | ? | 1996– | ![]() | University of Wisconsin–Madison | 0.45 m/0.4 m | 0.18 T | 0.3 MA | Extremely low aspect ratio | ![]() |
NSTX (National Spherical Torus Experiment)[26] | Operational | 1999– | ![]() | Princeton Plasma Physics Laboratory | 0.85 m/0.68 m | 0.3 T | 2 MA | Study the spherical tokamak concept | ![]() | |
Globus-M (UNU Globus-M)[27] | Operational | 1999– | ![]() | Ioffe Institute | 0.36 m/0.24 m | 0.4 T | 0.3 MA | Study the spherical tokamak concept | ||
ET (Electric Tokamak) | Recycled →ETPD | 1998 | 1999–2006 | ![]() | UCLA | 5 m/1 m | 0.25 T | 0.045 MA | Largest tokamak of its time | ![]() |
TCABR (Tokamak Chauffage Alfvén Brésilien) | Operational | 1980–1999 | 1999– | ![]() ![]() |
University of Sao Paulo | 0.615 m / 0.18 m | 1.1 T | 0.10 MA | Most important tokamak in the southern hemisphere | ![]() |
CDX-U (Current Drive Experiment-Upgrade) | Recycled →LTX | 2000–2005 | ![]() | Princeton Plasma Physics Laboratory | 0.3 m/? | 0.23 T | 0.03 MA | Study Lithium in plasma walls | ![]() | |
MAST (Mega-Ampere Spherical Tokamak)[28] | Recycled →MAST-Upgrade | 1997–1999 | 2000–2013 | ![]() | United Kingdom Atomic Energy Authority | 0.85 m/0.65 m | 0.55 T | 1.35 MA | Investigate spherical tokamak for fusion | ![]() |
HL-2A (Huan-Liuqi-2A) | Operational | 2000–2002 | 2002–2018 | ![]() | Southwestern Institute of Physics | 1.65 m/0.4 m | 2.7 T | 0.43 MA | H-mode physics, ELM mitigation | |
SST-1 (Steady State Superconducting Tokamak)[29] | Operational | 2001– | 2005– | ![]() | Institute for Plasma Research | 1.1 m/0.2 m | 3 T | 0.22 MA | Produce a 1000 s elongated double null divertor plasma | |
EAST (Experimental Advanced Superconducting Tokamak)[30] | Operational | 2000–2005 | 2006– | ![]() | Hefei Institutes of Physical Science | 1.85 m/0.43 m | 3.5 T | 0.5 MA | Superheated plasma for over 101 s at 120 M°C and 20 s at 160 M°C[31] | ![]() |
J-TEXT (Joint TEXT) | Operational | TEXT (Texas EXperimental Tokamak) | 2007– | ![]() | Huazhong University of Science and Technology | 1.05 m/0.26 m | 2.0 T | 0.2 MA | Develop plasma control | |
KSTAR (Korea Superconducting Tokamak Advanced Research)[32] | Operational | 1998–2007 | 2008– | ![]() | National Fusion Research Institute | 1.8 m/0.5 m | 3.5 T | 2 MA | Tokamak with fully superconducting magnets, 48 s-long operation at 100 MK[33] | ![]() |
LTX (Lithium Tokamak Experiment) | Operational | 2005–2008 | 2008– | ![]() | Princeton Plasma Physics Laboratory | 0.4 m/? | 0.4 T | 0.4 MA | Study Lithium in plasma walls | ![]() |
QUEST (Q-shu University Experiment with Steady-State Spherical Tokamak)[34] | Operational | 2008– | ![]() | Kyushu University | 0.68 m/0.4 m | 0.25 T | 0.02 MA | Study steady state operation of a Spherical Tokamak | ![]() | |
Kazakhstan Tokamak for Material testing (KTM) | Operational | 2000–2010 | 2010– | ![]() | National Nuclear Center of the Republic of Kazakhstan | 0.86 m/0.43 m | 1 T | 0.75 MA | Testing of wall and divertor | |
ST25-HTS[35] | Operational | 2012–2015 | 2015– | ![]() | Tokamak Energy Ltd | 0.25 m/0.125 m | 0.1 T | 0.02 MA | Steady state plasma | ![]() |
WEST (Tungsten Environment in Steady-state Tokamak) | Operational | 2013–2016 | 2016– | ![]() | Département de Recherches sur la Fusion Contrôlée | 2.5 m/0.5 m | 3.7 T | 1 MA | Superconducting tokamak with active cooling | ![]() |
ST40[36] | Operational | 2017–2018 | 2018– | ![]() | Tokamak Energy Ltd | 0.4 m/0.3 m | 3 T | 2 MA | First high field spherical tokamak | ![]() |
MAST-U (Mega-Ampere Spherical Tokamak Upgrade)[37] | Operational | 2013–2019 | 2020– | ![]() | United Kingdom Atomic Energy Authority | 0.85 m/0.65 m | 0.92 T | 2 MA | Test new exhaust concepts for a spherical tokamak | |
HL-2M (Huan-Liuqi-2M)[38] | Operational | 2018–2019 | 2020– | ![]() | Southwestern Institute of Physics | 1.78 m/0.65 m | 2.2 T | 1.2 MA | Elongated plasma with 200 MK | ![]() |
JT-60SA (Japan Torus-60 super, advanced)[39] | Operational | 2013–2020 | 2021– | ![]() | Japan Atomic Energy Research Institute | 2.96 m/1.18 m | 2.25 T | 5.5 MA | Optimise plasma configurations for ITER and DEMO with full non-inductive steady-state operation | ![]() |
T-15MD | Operational | 2010–2020 | 2021– | ![]() | Kurchatov Institute | 1.48 m/0.67 m | 2 T | 2 MA | Hybrid fusion/fission reactor | ![]() |
ITER[40] | Under construction | 2013–2025? | 2025? | ![]() | ITER Council | 6.2 m/2.0 m | 5.3 T | 15 MA ? | Demonstrate feasibility of fusion on a power-plant scale with 500 MW fusion power | ![]() |
SPARC[41][42][43][44][45] | Under construction | 2021– | 2025 | ![]() | Commonwealth Fusion Systems and MIT Plasma Science and Fusion Center | 1.85 m/0.57 m | 12.2 T | 8.7 MA | Compact, high-field tokamak with ReBCO coils and 100 MW planned fusion power | ![]() |
DTT (Divertor Tokamak Test facility)[46][47] | Planned | 2022–2027? | 2027? | ![]() | ENEA | 2.14 m/0.70 m | 6 T ? | 5.5 MA ? | Superconducting tokamak to study power exhaust | |
SST-2 (Steady State Tokamak-2)[48] | Planned | 2027? | ![]() | Institute for Plasma Research | 4.42 m/1.47 m | 5.42 T | 11.2 MA | Full-fledged fusion reactor with tritium breeding and up to 500 MW output | ||
CFETR (China Fusion Engineering Test Reactor)[49] | Planned | ≥2023 | 2030? | ![]() | Institute of Plasma Physics, Chinese Academy of Sciences | 7.2 m/2.2 m ? | 6.5 T ? | 14 MA ? | Bridge gaps between ITER and DEMO, planned fusion power 1000 MW | |
ST-F1 (Spherical Tokamak - Fusion 1)[50] | Planned | 2027? | ![]() | Tokamak Energy Ltd | 1.4 m/0.8 m ? | 4 T | 5 MA | Spherical tokamak with Q=3 and hundreds of MW planned electrical output | ||
STEP (Spherical Tokamak for Energy Production) | Planned | 2032-2040 | 2040 D-D Mid 2040s DT Campaign | ![]() | United Kingdom Atomic Energy Authority | 3 m/2 m ? | ? | 16.5 MA ? | Spherical tokamak with 100MW planned electrical output | [51] |
IGNITOR[52] | Planned[53] | ? | ? | ![]() | ENEA | 1.32 m/0.47 m | 13 T | 11 MA ? | Compact fusion reactor with self-sustained plasma and 100 MW of planned fusion power | |
JA-DEMO | Planned | 2030? | 2050? | ![]() |
? | 8.5 m/2.4 m[54] | 5.94 T | 12.3 MA | Prototype for development of Commercial Fusion Reactors 1.5-2GW Fusion output.[55] | |
K-DEMO (Korean fusion demonstration tokamak reactor)[56] | Planned | 2037? | ![]() | National Fusion Research Institute | 6.8 m/2.1 m | 7 T | 12 MA ? | Prototype for the development of commercial fusion reactors with around 2200 MW of fusion power | ![]() | |
DEMO (DEMOnstration Power Station) | Planned | 2040? | 2050? | ? | 9 m/3 m ? | 6 T ? | 20 MA ? | Prototype for a commercial fusion reactor | ![]() |
Stellarator
Device name | Status | Construction | Operation | Type | Location | Organisation | Major/minor radius | B-field | Purpose | Image |
---|---|---|---|---|---|---|---|---|---|---|
Model A | Shut down | 1952–1953 | 1953–? | Figure-8 | ![]() | Princeton Plasma Physics Laboratory | 0.3 m/0.02 m | 0.1 T | First stellarator, table-top device | |
Model B | Shut down | 1953–1954 | 1954–1959 | Figure-8 | ![]() | Princeton Plasma Physics Laboratory | 0.3 m/0.02 m | 5 T | Development of plasma diagnostics | |
Model B-1 | Shut down | ?–1959 | Figure-8 | ![]() | Princeton Plasma Physics Laboratory | 0.25 m/0.02 m | 5 T | Yielded 1 MK plasma temperatures, showed cooling by X-ray radiation from impurities | ||
Model B-2 | Shut down | 1957 | Figure-8 | ![]() | Princeton Plasma Physics Laboratory | 0.3 m/0.02 m | 5 T | Electron temperatures up to 10 MK | ||
Model B-3 | Shut down | 1957 | 1958– | Figure-8 | ![]() | Princeton Plasma Physics Laboratory | 0.4 m/0.02 m | 4 T | Last figure-8 device, confinement studies of ohmically heated plasma | |
Model B-64 | Shut down | 1955 | 1955 | Square | ![]() | Princeton Plasma Physics Laboratory | ? m/0.05 m | 1.8 T | ||
Model B-65 | Shut down | 1957 | 1957 | Racetrack | ![]() | Princeton Plasma Physics Laboratory | ||||
Model B-66 | Shut down | 1958 | 1958–? | Racetrack | ![]() | Princeton Plasma Physics Laboratory | ||||
Wendelstein 1-A | Shut down | 1960 | Racetrack | ![]() | Max-Planck-Institut für Plasmaphysik | 0.35 m/0.02 m | 2 T | ℓ=3 showed that stellarators can overcome Bohm diffusion, "Munich mystery" | ||
Wendelstein 1-B | Shut down | 1960 | Racetrack | ![]() | Max-Planck-Institut für Plasmaphysik | 0.35 m/0.02 m | 2 T | ℓ=2 | ||
Model C | Recycled →ST | 1957–1961 | 1961–1969 | Racetrack | ![]() | Princeton Plasma Physics Laboratory | 1.9 m/0.07 m | 3.5 T | Suffered from large plasma losses by Bohm diffusion through "pump-out" | |
L-1 | Shut down | 1963 | 1963–1971 | round | ![]() | Lebedev Physical Institute | 0.6 m/0.05 m | 1 T | First Soviet stellarator, overcame Bohm diffusion | |
SIRIUS | Shut down | 1964–? | Racetrack | ![]() | Kharkiv Institute of Physics and Technology (KIPT) | |||||
TOR-1 | Shut down | 1967 | 1967–1973 | ![]() | Lebedev Physical Institute | 0.6 m/0.05 m | 1 T | |||
TOR-2 | Shut down | ? | 1967–1973 | ![]() | Lebedev Physical Institute | 0.63 m/0.036 m | 2.5 T | |||
Uragan-1 | Shut down | 1960–1967 | 1967–? | Racetrack | ![]() | National Science Center, Kharkiv Institute of Physics and Technology (NSC KIPT) | 1.1 m/0.1 m | 1 T | Overcame Bohm-diffusion by a factor of 30 | |
CLASP (Closed Line And Single Particle)[57] | Shut down | ? | 1967–? | ![]() | United Kingdom Atomic Energy Authority | 0.3 m/0.056 m | 0.1 T | Study confinement of electrons in a high-shear stellarator | ||
TWIST[57] | Shut down | ? | 1967–? | ![]() | United Kingdom Atomic Energy Authority | 0.32 m/0.045 m | 0.3 T | Study turbulent heating | ||
Proto-CLEO[57] | Shut down | ? | 1968–? | single-turn helical winding inside toroidal field conductors | ![]() ![]() | United Kingdom Atomic Energy Authority | 0.4 m/0.05 m | 0.5 T | confirmed plasma confinement times of neoclassical theory | |
TORSO[57] | Shut down | ? | 1972–? | Ultimate torsatron | ![]() | United Kingdom Atomic Energy Authority | 0.4 m/0.05 m | 2 T | ||
CLEO[57] | Shut down | ? | 1974–? | ![]() | United Kingdom Atomic Energy Authority | 0.9 m/0.125 m | 2 T | Study of particle transport and beta limits, reached similar performance as tokamaks | ||
Wendelstein 2-A | Shut down | 1965–1968 | 1968–1974 | Heliotron | ![]() | Max-Planck-Institut für Plasmaphysik | 0.5 m/0.05 m | 0.6 T | Good plasma confinement | ![]() |
Saturn[58] | Shut down | 1970 | 1970–? | Torsatron | ![]() | Kharkiv Institute of Physics and Technology | 0.36 m/0.08 m | 1 T | first Torsatron, ℓ=3, m=8 field periods, base for several torsatrons at KIPT | |
Wendelstein 2-B | Shut down | ?–1970 | 1971–? | Heliotron | ![]() | Max-Planck-Institut für Plasmaphysik | 0.5 m/0.055 m | 1.25 T | Demonstrated similar performance as tokamaks | ![]() |
Vint-20[59] | Shut down | 1972 | 1973–? | Torsatron | ![]() | Kharkiv Institute of Physics and Technology | 0.315 m/0.0725 m | 1.8 T | single-pole ℓ=1, m=13 field periods | |
L-2 | Shut down | ? | 1975–? | ![]() | Lebedev Physical Institute | 1 m/0.11 m | 2.0 T | |||
WEGA (Wendelstein Experiment in Greifswald für die Ausbildung) | Recycled →HIDRA | 1972–1975 | 1975–2013 | Classical stellarator | ![]() | Max-Planck-Institut für Plasmaphysik | 0.72 m/0.15 m | 1.4 T | Test lower hybrid heating | ![]() |
Wendelstein 7-A | Shut down | ? | 1975–1985 | Classical stellarator | ![]() | Max-Planck-Institut für Plasmaphysik | 2 m/0.1 m | 3.5 T | First "pure" stellarator without plasma current, solved stellarator heating problem | |
Heliotron-E | Shut down | ? | 1980–? | Heliotron | ![]() | 2.2 m/0.2 m | 1.9 T | |||
Heliotron-DR | Shut down | ? | 1981–? | Heliotron | ![]() | 0.9 m/0.07 m | 0.6 T | |||
Uragan-3 (M [uk])[60] | Operational | ? | 1982–?[61] M: 1990– | Torsatron | ![]() | National Science Center, Kharkiv Institute of Physics and Technology (NSC KIPT) | 1.0 m/0.12 m | 1.3 T | ? | |
Auburn Torsatron (AT) | Shut down | ? | 1984–1990 | Torsatron | ![]() | Auburn University | 0.58 m/0.14 m | 0.2 T | ![]() | |
Wendelstein 7-AS | Shut down | 1982–1988 | 1988–2002 | Modular, advanced stellarator | ![]() | Max-Planck-Institut für Plasmaphysik | 2 m/0.13 m | 2.6 T | First computer-optimized stellarator, first H-mode in a stellarator in 1992 | ![]() |
Advanced Toroidal Facility (ATF) | Shut down | 1984–1988[62] | 1988–1994 | Torsatron | ![]() | Oak Ridge National Laboratory | 2.1 m/0.27 m | 2.0 T | First large American stellarator after Tokamak stampede, high-beta operation, >1h plasma operation | ![]() |
Compact Helical System (CHS) | Shut down | ? | 1989–? | Heliotron | ![]() | National Institute for Fusion Science | 1 m/0.2 m | 1.5 T | ||
Compact Auburn Torsatron (CAT) | Shut down | ?–1990 | 1990–2000 | Torsatron | ![]() | Auburn University | 0.53 m/0.11 m | 0.1 T | Study magnetic flux surfaces | ![]() |
H-1 (Heliac-1)[63] | Operational | 1992– | Heliac | ![]() ![]() | Research School of Physical Sciences and Engineering, Australian National University | 1.0 m/0.19 m | 0.5 T | shipped to China in 2017 | ![]() | |
TJ-K (Tokamak de la Junta Kiel)[64] | Operational | TJ-IU (1999) | 1994– | Torsatron | ![]() | University of Stuttgart | 0.60 m/0.10 m | 0.5 T | One helical and two vertical coil sets; Teaching; moved from Kiel to Stuttgart in 2005 | |
TJ-II (Tokamak de la Junta II)[65] | Operational | 1991–1996 | 1997– | flexible Heliac | ![]() | National Fusion Laboratory, Centro de Investigaciones Energéticas, Medioambientales y Tecnológicas | 1.5 m/0.28 m | 1.2 T | Study plasma in flexible configuration | ![]() |
LHD (Large Helical Device)[66] | Operational | 1990–1998 | 1998– | Heliotron | ![]() | National Institute for Fusion Science | 3.5 m/0.6 m | 3 T | Demonstrated long-term operation of large superconducting coils | ![]() |
HSX (Helically Symmetric Experiment)[67] | Operational | 1999– | Modular, quasi-helically symmetric | ![]() | University of Wisconsin–Madison | 1.2 m/0.15 m | 1 T | Investigate plasma transport in quasi-helically-symmetric field, similar to tokamaks | ![]() | |
Heliotron J[68] | Operational | 2000– | Heliotron | ![]() | Institute of Advanced Energy | 1.2 m/0.1 m | 1.5 T | Study helical-axis heliotron configuration | ||
Columbia Non-neutral Torus (CNT) | Operational | ? | 2004– | Circular interlocked coils | ![]() | Columbia University | 0.3 m/0.1 m | 0.2 T | Study of non-neutral (mostly electron) plasmas | |
Uragan-2(M)[60] | Operational | 1988–2006 | 2006–[69] | Heliotron, Torsatron | ![]() | National Science Center, Kharkiv Institute of Physics and Technology (NSC KIPT) | 1.7 m/0.22 m | 2.4 T | ℓ=2 Torsatron | |
Quasi-poloidal stellarator (QPS)[70][71] | Cancelled | 2001–2007 | – | Modular | ![]() | Oak Ridge National Laboratory | 0.9 m/0.33 m | 1.0 T | Stellarator research | ![]() |
NCSX (National Compact Stellarator Experiment) | Cancelled | 2004–2008 | – | Helias | ![]() | Princeton Plasma Physics Laboratory | 1.4 m/0.32 m | 1.7 T | High-β stability | ![]() |
Compact Toroidal Hybrid (CTH) | Operational | ? | 2007?– | Torsatron | ![]() | Auburn University | 0.75 m/0.2 m | 0.7 T | Hybrid stellarator/tokamak | ![]() |
HIDRA (Hybrid Illinois Device for Research and Applications)[72] | Operational | 2013–2014 (WEGA) | 2014– | ? | ![]() | University of Illinois | 0.72 m/0.19 m | 0.5 T | Stellarator and tokamak in one device, capable of long pulse steady-state operation; study plasma-wall interactions | ![]() |
UST_2[73] | Operational | 2013 | 2014– | modular three period quasi-isodynamic | ![]() | Charles III University of Madrid | 0.29 m/0.04 m | 0.089 T | 3D-printed stellarator | ![]() |
Wendelstein 7-X[74] | Operational | 1996–2022 | 2015– | Helias | ![]() | Max-Planck-Institut für Plasmaphysik | 5.5 m/0.53 m | 3 T | Steady-state plasma in large fully optimized stellarator | ![]() |
SCR-1 (Stellarator of Costa Rica) | Operational | 2011–2015 | 2016– | Modular | ![]() | Costa Rica Institute of Technology | 0.14 m/0.042 m | 0.044 T | ![]() | |
MUSE[75] | Operational | 2022–2023 | 2023– | Quasiaxi-symmetrical | ![]() |
Princeton Plasma Physics Laboratory | 0.3 m/0.075 m | 0.15 T | First stellarator with permanent magnets | ![]() |
CFQS (Chinese First Quasi-Axisymmetric Stellarator)[76] | Under construction | 2017– | Helias | ![]() |
Southwest Jiaotong University, National Institute for Fusion Science in Japan | 1 m/0.25 m | 1 T | m=2 quasi-axisymmetric stellarator, modular | ![]() | |
EFPP (European Fusion Power Plant)[77] | Planned | 2030 ? | 2045 ? | Helias | ![]() |
Gauss Fusion | 7–9 T ? | Fusion power plant with 2–3 GW output |
Magnetic mirror
- Tabletop/Toytop, Lawrence Livermore National Laboratory, Livermore CA.
- DCX/DCX-2, Oak Ridge National Laboratory
- OGRA (Odin GRAm neitronov v sutki, one gram of neutrons per day), Akademgorodok, Russia. A 20-meter-long pipe
- Baseball I/Baseball II Lawrence Livermore National Laboratory, Livermore CA.
- 2X/2XIII/2XIII-B, Lawrence Livermore National Laboratory, Livermore CA.
- TMX, TMX-U Lawrence Livermore National Laboratory, Livermore CA.
- MFTF Lawrence Livermore National Laboratory, Livermore CA.
- Gas Dynamic Trap at Budker Institute of Nuclear Physics, Akademgorodok, Russia.
Toroidal Z-pinch
- Perhapsatron (1953, USA)
- ZETA (Zero Energy Thermonuclear Assembly) (1957, United Kingdom)
Reversed field pinch (RFP)
- ETA-BETA II in Padua, Italy (1979–1989)
- RFX (Reversed-Field eXperiment), Consorzio RFX, Padova, Italy[78]
- MST (Madison Symmetric Torus), University of Wisconsin–Madison, United States[79]
- T2R, Royal Institute of Technology, Stockholm, Sweden
- TPE-RX, AIST, Tsukuba, Japan
- KTX (Keda Torus eXperiment) in China (since 2015)[80]
Spheromak
Field-reversed configuration (FRC)
- C-2 Tri Alpha Energy
- C-2U Tri Alpha Energy
- C-2W TAE Technologies
- LSX University of Washington
- IPA University of Washington
- HF University of Washington
- IPA- HF University of Washington
Other toroidal machines
- TMP (Tor s Magnitnym Polem, torus with magnetic field): A porcelain torus with major radius 80 cm, minor radius 13 cm, toroidal field of 1.5 T and plasma current 0.25 MA, predecessor to the first tokamak (1955, USSR)
Open field lines
Plasma pinch
- Trisops – 2 facing theta-pinch guns
- FF-2B, Lawrenceville Plasma Physics, United States[81]
Levitated dipole
- Levitated Dipole Experiment (LDX), MIT/Columbia University, United States[82]
Laser-driven
Device name | Status | Construction | Operation | Description | Peak laser power | Pulse energy | Fusion yield | Location | Organisation | Image |
---|---|---|---|---|---|---|---|---|---|---|
4 pi laser | Shut down | 196? | Semiconductor laser | 5 GW | 12 J | ![]() | LLNL | |||
Long path laser | Shut down | 1972 | 1972 | First ICF laser with neodymium doped glass (Nd:glass) as lasing medium | 5 GW | 50 J | ![]() | LLNL | ||
Single Beam System (SBS) "67" | Shut down | 1971-1973 | 1973 | Single-beam CO2 laser[83] | 200 GW | 1 kJ | ![]() | LANL | ||
Double Bounce Illumination System (DBIS) | Shut down | 1972-1974 | 1974-1990 | First private laser fusion effort, YAG laser, neutron yield 104 to 3×105 neutrons | 1 kJ | ≈100 nJ | ![]() | KMS Fusion | ||
MERLIN (Medium Energy Rod Laser Incorporating Neodymium), N78 laser | Shut down | 1972-1975 | 1975-? | Nd:glass laser | 100 GW | 40 J | ![]() | AWE | ||
Cyclops laser | Shut down | 1975 | 1975 | Single-beam Nd:glass laser, prototype for Shiva[84] | 1 TW | 270 J | ![]() | LLNL | ||
Janus laser | Shut down | 1974-1975 | 1975 | Two-beam Nd:glass laser demonstrated laser compression and thermonuclear burn of deuterium–tritium | 1 TW | 10 J | ![]() | LLNL | ||
Gemini laser, Dual-Beam Module (DBM) | Shut down | ≤ 1975 | 1976 | Two-beam CO2 laser, tests for Helios | 5 TW | 2.5 kJ | ![]() | LANL | ||
Argus laser | Shut down | 1976 | 1976-1981 | Two-beam Nd:glass laser, advanced the study of laser-target interaction and paved the way for Shiva | 4 TW | 2 kJ | ≈3 mJ | ![]() | LLNL | |
Vulcan laser (Versicolor Ultima Lux Coherens pro Academica Nostra)[85] | Operational | 1976-1977 | 1977- | 8-beam Nd:glass laser, highest-intensity focussed laser in the world in 2005[86] | 1 PW | 2.6 kJ | ![]() | RAL | ||
Shiva laser | Shut down | 1977 | 1977-1981 | 20-beam Nd:glass laser; proof-of-concept for Nova; fusion yield of 1011 neutrons; found that its infrared wavelength of 1062 nm was too long to achieve ignition | 30 TW | 10.2 kJ | ≈0.1 J | ![]() | LLNL | |
Helios laser, Eight-Beam System (EBS) | Shut down | 1975-1978 | 1978 | 8-beam CO2 laser; Media at Wikimedia Commons | 20 TW | 10 kJ | ![]() | LANL | ||
HELEN (High Energy Laser Embodying Neodymium) | Shut down | 1976-1979 | 1979-2009 | Two-beam Nd:glass laser | 1 TW | 200 J | ![]() | RAL | ||
ISKRA-4 | Operational | -1979 | 1979- | 8-beam iodine gas laser, prototype for ISKRA-5[87] | 10 TW | 2 kJ | 6 mJ | ![]() | RFNC-VNIIEF | |
Sprite laser[85] | Shut down | 1981-1983 | 1983-1995 | First high-power Krypton fluoride laser used for target irradiation, λ=249 nm | 1 TW | 7.5 J | ![]() | RAL | ||
Gekko XII | Operational | 1983- | 12-beam, Nd:glass laser | 500 TW | 10 kJ | ![]() | Institute for Laser Engineering | |||
Novette laser | Shut down | 1981-1983 | 1983-1984 | Nd:glass laser to validate the Nova design, first X-ray laser[88] | 13 TW | 18 kJ | ![]() | LLNL | ||
Antares laser, High Energy Gas Laser Facility (HEGLF) | Shut down | 1983[89] | 24-beam largest CO2 laser ever built. Missed goal of scientific fusion breakeven, because production of hot electrons in target plasma due to long 10.6 μm wavelength of laser resulted in poor laser/plasma energy coupling[88] | 200 TW | 40 kJ | ![]() | LANL | |||
PHAROS laser | Operational | 198? | Two-beam Nd:glass laser | 300 GW | 1 kJ | ![]() | NRL | |||
Nova laser | Shut down | 1984-1999 | 10-beam NIR and frequency-tripled 351 nm UV laser; fusion yield of 1013 neutrons; attempted ignition, but failed due to fluid instability of targets; led to construction of NIF | 1.3 PW | 120 kJ | 30 J | ![]() | LLNL | ||
ISKRA-5 | Operational | -1989 | 12-beam iodine gas laser, fusion yield 1010 to 1011 neutrons[87] | 100 TW | 30 kJ | 0.3 J | ![]() | RFNC-VNIIEF | ||
Aurora laser | Shut down | ≤ 1988-1989 | 1990 | 96-beam Krypton fluoride laser | ≈300 GW | 1.3 kJ | ![]() | LANL | ||
PALS, formerly "Asterix IV" | Operational | -1991 | 1991- | Iodine gas laser, λ=1315 nm | 3 TW | 1 kJ | ![]() ![]() | MPQ, CAS | ||
Trident laser | Operational | 198?-1992 | 1992-2017 | 3-beam Nd:glass laser; 2 x 400 J beams, 100 ps – 1 us; 1 beam ~100 J, 600 fs – 2 ns | 200 TW | 500 J | ![]() | LANL | ||
Nike laser | Operational | ≤ 1991-1994 | 1994- | 56-beam, most-capable Krypton fluoride laser for laser target interactions[90][91] | 2.6 TW | 3 kJ | ![]() | NRL | ||
OMEGA laser | Operational | ?-1995 | 1995- | 60-beam UV frequency-tripled Nd:glass laser, fusion yield 1014 neutrons | 60 TW | 40 kJ | 300 J | ![]() | LLE | |
Electra | Operational | Krypton fluoride laser, 5 Hz operation with 90,000+ shots continuous | 4 GW | 730 J | ![]() | NRL | ||||
LULI2000 | Operational | ? | 2003- | 6-beam Nd:glass laser, λ=1.06 μm, λ=0.53 μm, λ=0.26 μm | 500 GW | 600 J | ![]() | École polytechnique | ||
OMEGA EP | Operational | 2008- | 60-beam UV | 1.4 PW | 5 kJ | ![]() | LLE | |||
National Ignition Facility (NIF) | Operational | 1997-2009 | 2010- | 192-beam Nd:glass laser, achieved scientific breakeven with fusion gain of 1.5 and 1.2×1018 neutrons[92] | 500 TW | 2.05 MJ | 3.15 MJ | ![]() | LLNL | |
Orion | Operational | 2006-2010 | 2010- | 10-beams, λ=351 nm | 200 TW | 5 kJ | ![]() | AWE | ||
Laser Mégajoule (LMJ) | Operational | 1999-2014 | 2014- | Second-largest laser fusion facility, 10 out of 22 beam lines operational in 2022[93] | 800 TW | 1 MJ | ![]() | CEA | ||
Laser for Fast Ignition Experiments (LFEX) | Operational | 2003-2015 | 2015- | High-contrast heating laser for FIREX, λ=1053 nm | 2 PW | 10 kJ | 100 μJ | ![]() | Institute for Laser Engineering | |
HiPER (High Power Laser Energy Research Facility) | Cancelled | 2007-2015 | - | Pan-European project to demonstrate the technical and economic viability of laser fusion for the production of energy[94] | (4 PW) | (270 kJ) | (25 MJ) | ![]() | ||
Laser Inertial Fusion Energy (LIFE) | Cancelled | 2008-2013 | - | Effort to develop a fusion power plant succeeding NIF | (2.2 MJ) | (40 MJ) | ![]() | LLNL | ||
ISKRA-6 | Planned | ? | ? | 128 beam Nd:glass laser | 300 TW? | 300 kJ? | ![]() | RFNC-VNIIEF |
Z-pinch
- Z Pulsed Power Facility
- ZEBRA device at the University of Nevada's Nevada Terawatt Facility[95]
- Saturn accelerator at Sandia National Laboratory[96]
- MAGPIE at Imperial College London
- COBRA at Cornell University
- PULSOTRON[97]
- Z-FFR (Z(-pinch)-Fission-Fusion Reactor), a nuclear fusion–fission hybrid machine to be built in Chengdu, China by 2025 and generate power as early as 2028
- FRX-L
- FRCHX
- General Fusion – under development
- LINUS project
- Smirnov, V.P. (30 December 2009). "Tokamak foundation in USSR/Russia 1950–1990". Nuclear Fusion. 50 (1): 014003. doi:10.1088/0029-5515/50/1/014003. eISSN 1741-4326. ISSN 0029-5515. S2CID 17487157.
- Taylor, R. J.; Lee, P.; Luhmann, N. C. Jr (1981). ICRF heating, particle transport and fluctuations in tokamaks (PDF) (Report). Archived from the original (PDF) on 2022-02-25.
- Argenti, D.; Bonizzoni, G.; Cirant, S.; Corti, S.; Grosso, G.; Lampis, G.; Rossi, L.; Carretta, U.; Jacchia, A.; De Luca, F.; Fontanesi, M. (June 1981). "The Thor tokamak experiment". Il Nuovo Cimento B. 63 (2): 471–486. Bibcode:1981NCimB..63..471A. doi:10.1007/BF02755093. eISSN 1826-9877. S2CID 123205206.
- Robert Arnoux (2009-05-18). "From Russia with love".
- "Forschungszentrum Jülich – Plasmaphysik (IEK-4)". fz-juelich.de (in German).
- "Tokamak Fusion Test Reactor". 2011-04-26. Archived from the original on 2011-04-26.
- "EFDA-JET, the world's largest nuclear fusion research experiment". 2006-04-30. Archived from the original on 2006-04-30.
- ":::. Instituto Nacional de Investigaciones Nucleares | Fusión nuclear ". 2009-11-25. Archived from the original on 2009-11-25.
- "All-the-Worlds-Tokamaks". tokamak.info.
- Yoshikawa, M. (2006-10-02). "JT-60 Project". Fusion Technology 1978. 2: 1079. Bibcode:1979fute.conf.1079Y. Archived from the original on 2006-10-02.
- "diii-d:home [MFE: DIII-D and Theory]". fusion.gat.com. Retrieved 2018-09-04.
- "DIII-D National Fusion Facility (DIII-D) | U.S. DOE Office of Science (SC)". science.energy.gov. Retrieved 2018-09-04.
- "U of S". 2011-07-06. Archived from the original on 2011-07-06.
- "Tore Supra". www-fusion-magnetique.cea.fr. Retrieved 2018-09-04.
- "Tokamak Department, Institute of Plasma Physics". 2014-05-12. Archived from the original on 2014-05-12.
- "COMPASS – General information". 2013-10-25. Archived from the original on 2013-10-25.
- . 2006-04-24 https://web.archive.org/web/20060424061102/http://www.fusion.org.uk/culham/start.htm. Archived from the original on 2006-04-24.
{{cite web}}
: Missing or empty|title=
(help) - "MIT Plasma Science & Fusion Center: research>alcator>". 2015-07-09. Archived from the original on 2015-07-09.
- "Centro de Fusão Nuclear". cfn.ist.utl.pt. Archived from the original on 2010-03-07. Retrieved 2012-02-13.
- "Pegasus Toroidal Experiment". pegasus.ep.wisc.edu.
- "Globus-M experiment". globus.rinno.ru/ (in Russian). Retrieved 2021-10-23.
- "MAST – the Spherical Tokamak at UKAEA Culham". 2006-04-21. Archived from the original on 2006-04-21.
- "The SST-1 Tokamak Page". 2014-06-20. Archived from the original on 2014-06-20.
- "Chinese "Artificial Sun" experimental fusion reactor sets world record for superheated plasma time". The Nation. May 29, 2021.
- . 2008-05-30 https://web.archive.org/web/20080530221257/http://www.nfri.re.kr/. Archived from the original on 2008-05-30.
{{cite web}}
: Missing or empty|title=
(help) - McFadden, Christopher (29 March 2024). "South Korean 'artificial sun' reaches 7 times the Sun's core temperature". Interesting Engineering. Retrieved 30 March 2024.
- . 2013-11-10 https://web.archive.org/web/20131110043518/http://www.triam.kyushu-u.ac.jp/QUEST_HP/quest_e.doc. Archived from the original on 2013-11-10.
{{cite web}}
: Missing or empty|title=
(help) - "ST25 » Tokamak Energy". Archived from the original on 2019-03-26. Retrieved 2018-10-21.
- "ST40 » Tokamak Energy". Archived from the original on 2019-03-26. Retrieved 2018-10-21.
- "Status and Plans on MAST-U". 2016-12-13.
- "The JT-60SA project". www.jt60sa.org.
- Harris, Mark (October 4, 2023). "2023 Climate Tech Companies to Watch: Commonwealth and its compact tokamak". MIT Technology Review. Retrieved February 10, 2024.
- Creely, A. J.; Greenwald, M. J.; Ballinger, S. B.; Brunner, D.; Canik, J.; Doody, J.; Fülöp, T.; Garnier, D. T.; Granetz, R.; Gray, T. K.; Holland, C. (2020). "Overview of the SPARC tokamak". Journal of Plasma Physics. 86 (5). Bibcode:2020JPlPh..86e8602C. doi:10.1017/S0022377820001257. hdl:1721.1/136131. ISSN 0022-3778.
- Chesto, Jon (2021-03-03). "MIT energy startup homes in on fusion, with plans for 47-acre site in Devens". BostonGlobe.com. Retrieved 2021-03-03.
- Verma, Pranshu. Nuclear fusion power inches closer to reality. The Washington Post, August 26, 2022.
- "The DTT Project". Archived from the original on 2019-03-30. Retrieved 2020-02-21.
- "The new Divertor Tokamak Test facility" (PDF). Archived from the original (PDF) on 2020-02-21. Retrieved 2020-02-21.
- Srinivasan, R. (2016). "Design and analysis of SST-2 fusion reactor". Fusion Engineering and Design. 112: 240–243. Bibcode:2016FusED.112..240S. doi:10.1016/j.fusengdes.2015.12.044. ISSN 0920-3796.
- Zhuang, G.; Li, G.Q.; Li, J.; Wan, Y.X.; Liu, Y.; Wang, X.L.; Song, Y.T.; Chan, V.; Yang, Q.W.; Wan, B.N.; Duan, X.R.; Fu, P.; Xiao, B.J. (5 June 2019). "Progress of the CFETR design". Nuclear Fusion. 59 (11): 112010. Bibcode:2019NucFu..59k2010Z. doi:10.1088/1741-4326/ab0e27. eISSN 1741-4326. ISSN 0029-5515. S2CID 127585754.
- STEP, UKAEA. "STEP Project Partner Slide Deck". STEP UKAEA Portal. Retrieved 2023-04-04.
- "Ignited plasma in Tokamaks – The IGNITOR project". frascati.enea.it. Archived from the original on 2020-04-19.
- "CREMLIN WP2 Informal exchange meeting: The Russian-Italian IGNITOR Tokamak Project: Design and status of implementation". DESY-Konferenzverwaltung (Indico). 13 July 2017.
- Tobita, Kenji; Hiwatari, Ryoji; Sakamoto, Yoshiteru; Someya, Youji; Asakura, Nobuyuki; Utoh, Hiroyasu; Miyoshi, Yuya; Tokunaga, Shinsuke; Homma, Yuki; Kakudate, Satoshi; Nakajima, Noriyoshi; for Fusion DEMO, the Joint Special Design Team (2019-07-04). "Japan's Efforts to Develop the Concept of JA DEMO During the Past Decade". Fusion Science and Technology. 75 (5): 372–383. Bibcode:2019FuST...75..372T. doi:10.1080/15361055.2019.1600931. ISSN 1536-1055. S2CID 164357381.
- Iwai, Yasunori; Edao, Yuki; Kurata, Rie; Isobe, Kanetsugu (2021-05-01). "Basic concept of JA DEMO fuel cycle". Fusion Engineering and Design. 166: 112261. Bibcode:2021FusED.16612261I. doi:10.1016/j.fusengdes.2021.112261. ISSN 0920-3796. S2CID 233566366.
- Kim, K.; Im, K.; Kim, H. C.; Oh, S.; Park, J. S.; Kwon, S.; Lee, Y. S.; Yeom, J. H.; Lee, C. (2015). "Design concept of K-DEMO for near-term implementation". Nuclear Fusion. 55 (5): 053027. Bibcode:2015NucFu..55e3027K. doi:10.1088/0029-5515/55/5/053027. ISSN 0029-5515.
- Georgiyevskiy, A. V.; Solodovchenko, S. I.; Voitsenya, V. S. (13 February 2010). "Contributions of the "Saturn" to Modern Stellarator-Torsatron Research". Journal of Fusion Energy. 29 (4): 399–406. Bibcode:2010JFuE...29..399G. doi:10.1007/s10894-010-9284-0. eISSN 1572-9591. ISSN 0164-0313. S2CID 123305093.
- "History | ННЦ ХФТИ". kipt.kharkov.ua.
- "Uragan-3M | IPP NSC KIPT". ipp.kipt.kharkov.ua.
- "ORNL Review v17n3 1984.pdf | ORNL". www.ornl.gov.
- Department, Head of; [email protected]. "Plasma Research Laboratory – PRL – ANU". prl.anu.edu.au. Archived from the original on 2010-02-13. Retrieved 2005-12-26.
- "TJ-K – FusionWiki". fusionwiki.ciemat.es.
- CIEMAT. "Centro de Investigaciones Energéticas, Medioambientales y Tecnológicas". ciemat.es (in Spanish).
- "Large Helical Device Project". lhd.nifs.ac.jp. Archived from the original on 2010-04-12. Retrieved 2006-04-20.
- "HSX – Helically Symmetric eXperiment". hsx.wisc.edu.
- "Heliotron J Project". iae.kyoto-u.ac.jp/en/joint/heliotron-j.doc.
- "Uragan-2M | IPP NSC KIPT". ipp.kipt.kharkov.ua.
- "QPS Home Page". Archived from the original on 2016-04-24. Retrieved 2018-09-01.
- "Vying Fusion Energy - V. Queral". www.fusionvic.org.
- "Wendelstein 7-X". ipp.mpg.de/w7x.
- T.M. Qian, X. Chu, C. Pagano, D. Patch, M.C. Zarnstorff, B. Berlinger, D. Bishop, A. Chambliss, M. Haque, D. Seidita, C. Zhu (2023-10-31). "Design and construction of the MUSE permanent magnet stellarator". Journal of Plasma Physics. 89 (5): 955890502. Bibcode:2023JPlPh..89e9502Q. doi:10.1017/S0022377823000880.
{{cite journal}}
: CS1 maint: multiple names: authors list (link) - KINOSHITA, Shigeyoshi; SHIMIZU, Akihiro; OKAMURA, Shoichi; ISOBE, Mitsutaka; XIONG, Guozhen; LIU, Haifeng; XU, Yuhong; The CQFS Team (2019-06-03). "Engineering Design of the Chinese First Quasi-Axisymmetric Stellarator (CFQS)". Plasma and Fusion Research. 14: 3405097. Bibcode:2019PFR....1405097K. doi:10.1585/pfr.14.3405097. ISSN 1880-6821.
- "Introduction to the Gauss Fusion Initiative" (PDF). 2022-12-08.
- "CONSORZIO RFX – Ricerca Formazione Innovazione". igi.cnr.it. Archived from the original on 2009-09-01. Retrieved 2018-04-16.
- Hartog, Peter Den. "MST – UW Plasma Physics". plasma.physics.wisc.edu. Archived from the original on 2019-03-13. Retrieved 2013-02-28.
- Liu, Wandong; et, al. (2017). "Overview of Keda Torus eXperiment initial results". Nuclear Fusion. 57 (11): 116038. Bibcode:2017NucFu..57k6038L. doi:10.1088/1741-4326/aa7f21. ISSN 0029-5515. S2CID 116431906.
- "Report Oct 15, 2021" (PDF). 2021-10-15. Archived (PDF) from the original on 2021-10-25.
- "Levitated Dipole Experiment". 2004-08-23. Archived from the original on 2004-08-23.
- F Skoberne (July 1967). "Los Alamos Laser Fusion Program" (PDF).
- "Beam-propagation studies on Cyclops" (PDF). February 1976.
- Danson, Colin N.; et al. (2021). "A history of high-power laser research and development in the United Kingdom". High Power Laser Science and Engineering. 9. Bibcode:2021HPLSE...9E..18D. doi:10.1017/hpl.2021.5. eISSN 2052-3289. hdl:10044/1/89337. ISSN 2095-4719. S2CID 233401354.
- "RFNC-VNIIEF – Science – Laser physics". 2005-04-06. Archived from the original on 2005-04-06.
- Hora, Heinrich; Miley, George H, eds. (1984). Laser Interaction and Related Plasma Phenomena. Springer US. doi:10.1007/978-1-4615-7332-6. ISBN 978-1-4615-7334-0.
- Schwarzschild, Bertram M. (1984). "Fusion experiments have begun at Antares". Physics Today. 37 (9): 19. Bibcode:1984PhT....37i..19S. doi:10.1063/1.2916397.
- Lehecka, T.; Bodner, S.; Deniz, A. V.; Mostovych, A. N.; Obenschain, S. P.; Pawley, C. J.; Pronko, M. S. (December 1991). "The NIKE KrF laser fusion facility". Journal of Fusion Energy. 10 (4): 301–303. Bibcode:1991JFuE...10..301L. doi:10.1007/BF01052128. eISSN 1572-9591. ISSN 0164-0313. S2CID 122087249.
- Obenschain, Stephen; Lehmberg, Robert; Kehne, David; Hegeler, Frank; Wolford, Matthew; Sethian, John; Weaver, James; Karasik, Max; et al. (19 August 2015). "High-energy krypton fluoride lasers for inertial fusion". Applied Optics. 54 (31): F103-22. Bibcode:2015ApOpt..54F.103O. doi:10.1364/AO.54.00F103. eISSN 1539-4522. ISSN 0003-6935. PMID 26560597.
- CLERY, DANIEL (13 December 2022). "With historic explosion, a long sought fusion breakthrough". www.science.org. Retrieved 2022-12-14.
- "CEA – Laser Mégajoule". www-lmj.cea.fr.
- "The HiPER Project". Archived from the original on 2022-12-23.
- "University of Nevada, Reno. Nevada Terawatt Facility". archive.is. 2000-09-19. Archived from the original on 2000-09-19.
- "PULSOTRON". pulsotron.org. Archived from the original on 2019-04-01. Retrieved 2020-03-09.