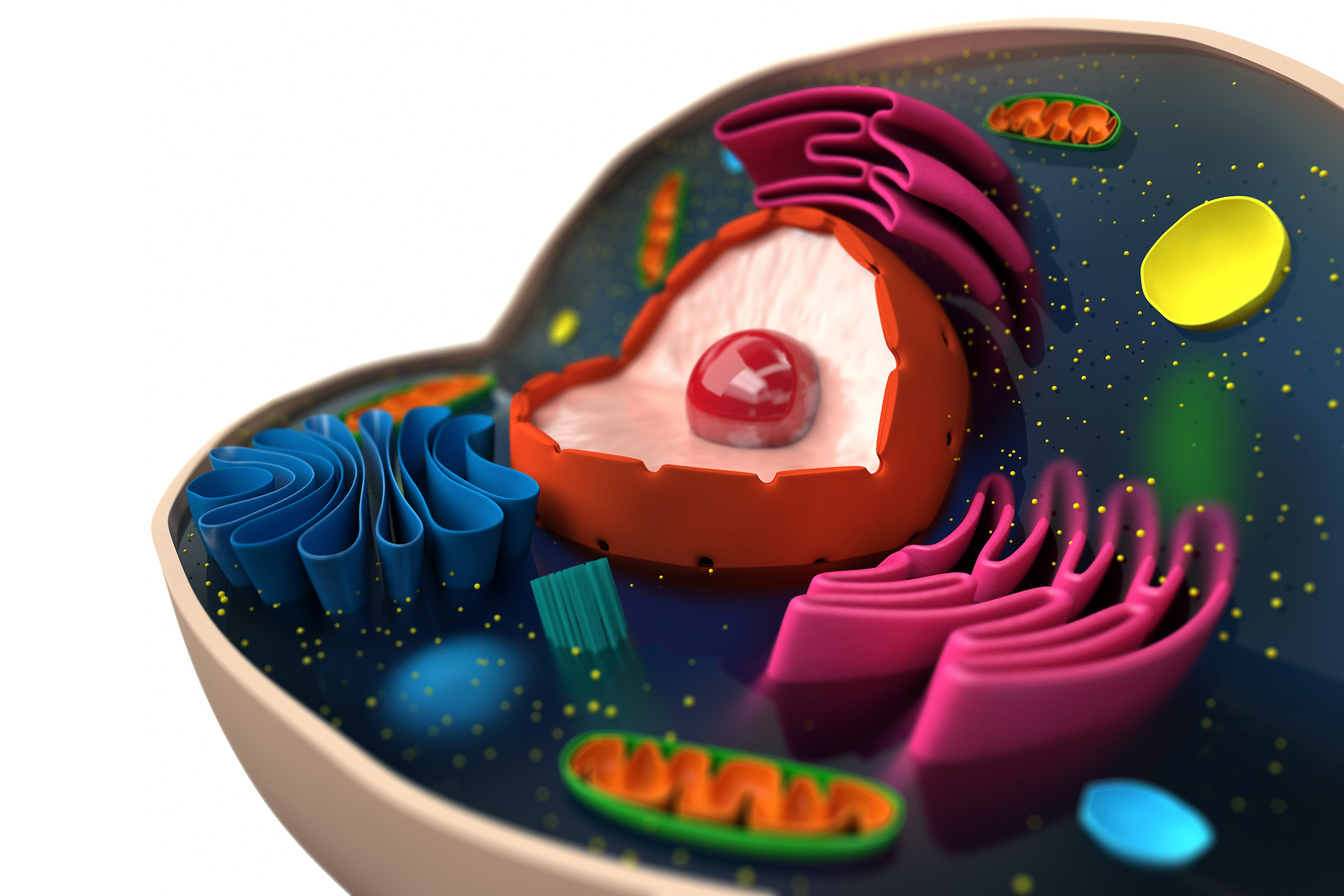
How cell identity is preserved when cells divide
MIT study suggests 3D folding of the genome is key to cells’ ability to store and pass on “memories” of which genes they should express.
Every cell in the human body contains the same genetic instructions, encoded in its DNA. However, out of about 30,000 genes, each cell expresses only those genes that it needs to become a nerve cell, immune cell, or any of the other hundreds of cell types in the body.
Each cell’s fate is largely determined by chemical modifications to the proteins that decorate its DNA; these modification in turn control which genes get turned on or off. When cells copy their DNA to divide, however, they lose half of these modifications, leaving the question: How do cells maintain the memory of what kind of cell they are supposed to be?
A new MIT study proposes a theoretical model that helps explain how these memories are passed from generation to generation when cells divide. The research team suggests that within each cell’s nucleus, the 3D folding of its genome determines which parts of the genome will be marked by these chemical modifications. After a cell copies its DNA, the marks are partially lost, but the 3D folding allows the cell to easily restore the chemical marks needed to maintain its identity. And each time a cell divides, chemical marks allow a cell to restore its 3D folding of its genome. This way, by juggling the memory between 3D folding and the marks, the memory can be preserved over hundreds of cell divisions.
“A key aspect of how cell types differ is that different genes are turned on or off. It's very difficult to transform one cell type to another because these states are very committed,” says Jeremy Owen PhD ’22, the lead author of the study. “What we have done in this work is develop a simple model that highlights qualitative features of the chemical systems inside cells and how they need to work in order to make memories of gene expression stable.”
Leonid Mirny, a professor in MIT’s Institute for Medical Engineering and Science and the Department of Physics, is the senior author of the paper , which appears today in Science . Dino Osmanović, a former postdoctoral fellow at MIT’s Center for the Physics of Living Systems, is also an author of the study.
Maintaining memory
Within the cell nucleus, DNA is wrapped around proteins called histones, forming a densely packed structure known as chromatin. Histones can display a variety of modifications that help control which genes are expressed in a given cell. These modifications generate “epigenetic memory,” which helps a cell to maintain its cell type. However, how this memory is passed on to daughter cells is somewhat of a mystery.
Previous work by Mirny’s lab has shown that the 3D structure of chromosomes is, to a great extent, determined by these epigenetic modifications, or marks. In particular, they found that certain chromatin regions, with marks telling cells not to read a particular segment of DNA, attract each other and form dense clumps called heterochromatin, which are difficult for the cell to access.
In their new study, Mirny and his colleagues wanted to answer the question of how those epigenetic marks are maintained from generation to generation. They developed a computational model of a polymer with a few marked regions, and saw that these marked regions collapse into each other, forming a dense clump. Then they studied how these marks are lost and gained.
When a cell copies its DNA to divide it between two daughter cells, each copy gets about half of the epigenetic marks. The cell then needs to restore the lost marks before the DNA is passed to the daughter cells, and the way chromosomes were folded serves as a blueprint for where these remaining marks should go.
These modifications are added by specialized enzymes known as “reader-writer” enzymes. Each of these enzymes is specific for a certain mark, and once they “read” existing marks, they “write” additional marks at nearby locations. If the chromatin is already folded into a 3D shape, marks will accumulate in regions that already had modifications inherited from the parent cell.
“There are several lines of evidence that suggest that the spreading can happen in 3D, meaning if there are two parts that are near each other in space, even if they're not adjacent along the DNA, then spreading can happen from one to another,” Owen says. “That is how the 3D structure can influence the spreading of these marks.”
This process is analogous to the spread of infectious disease, as the more contacts that a chromatin region has with other regions, the more likely it is to be modified, just as an individual is more likely to become infected as their number of contacts increases. In this analogy, dense regions of marked chromatin are like cities where people have many social interactions, while the rest of the genome is comparable to sparsely populated rural areas.
“That essentially means that the marks will be spreading in the dense region and will be very sparse anywhere outside it,” Mirny says.
The new model also suggests possible parallels between epigenetic memories stored in a folded polymer and memories stored in a neural network, he adds. Folding of marked regions can be thought of as analogous to the strong connections formed between neurons that fire together in a neural network.
“Broadly this suggests that akin to the way neural networks are able to do very complex information processing, the epigenetic memory mechanism we described may be able to process information, not only store it,” he says.
“One beautiful aspect of the work is how it offers and explores connections with ideas from the seemingly very distant corners of science, including spreading of infections (to describe formation of new chemical marks in the 3D vicinity of the existing one), associative memory in model neural networks, and protein folding,” says Alexander Grosberg, a professor of physics at New York University, who was not involved in the research.
Epigenetic erosion
While this model appeared to offer a good explanation for how epigenetic memory can be maintained, the researchers found that eventually, reader-writer enzyme activity would lead to the entire genome being covered in epigenetic modifications. When they altered the model to make the enzyme weaker, it didn’t cover enough of the genome and memories were lost in a few cell generations.
To get the model to more accurately account for the preservation of epigenetic marks, the researchers added another element: limiting the amount of reader-writer enzyme available. They found that if the amount of enzyme was kept between 0.1 and 1 percent of the number of histones (a percentage based on estimates of the actual abundance of these enzymes), their model cells could accurately maintain their epigenetic memory for up to hundreds of generations, depending on the complexity of the epigenetic pattern.
It is already known that cells begin to lose their epigenetic memory as they age, and the researchers now plan to study whether the process they described in this paper might play a role in epigenetic erosion and loss of cell identity. They also plan to model a disease called progeria, in which cells have a genetic mutation that leads to loss of heterochromatin. People with this disease experience accelerated aging.
“The mechanistic link between these mutations and the epigenetic changes that eventually happen is not well understood,” Owen says. “It would be great to use a model like ours where there are dynamic marks, together with polymer dynamics, to try and explain that.”
The researchers also hope to work with collaborators to experimentally test some of the predictions of their model, which could be done, for example, by altering the level of reader-writer enzymes in living cells and measuring the effect on epigenetic memory.
The research was funded by the National Human Genome Research Institute, the National Institute of General Medical Sciences, and the National Science Foundation.
Reprinted with permission of MIT News