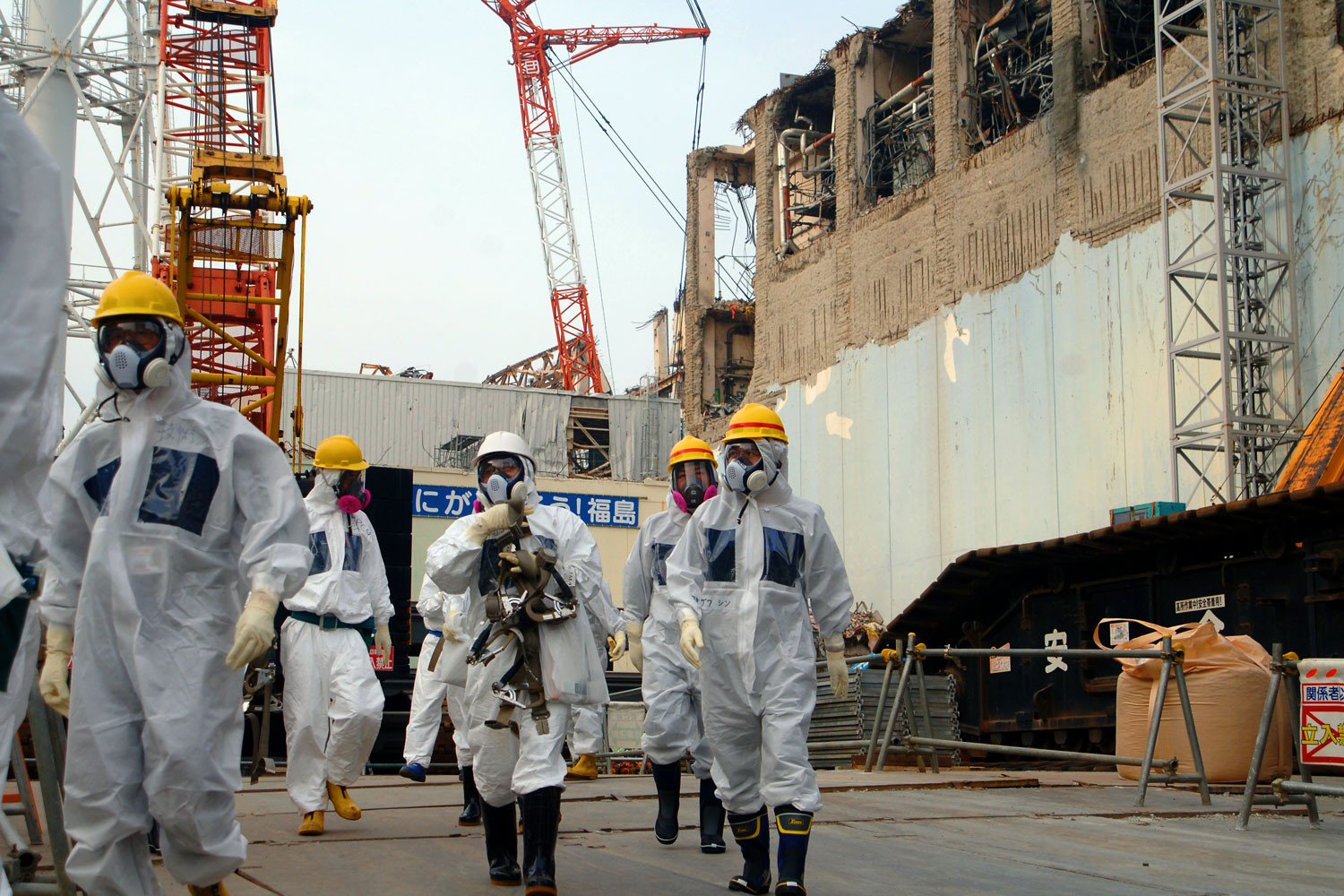
Lessons from Fukushima: Prepare for the unlikely
An analysis of the 2011 nuclear accident reveals a need for more preparation, training, and protocols for responding to low-probability accidents.
When a devastating earthquake and tsunami overwhelmed the protective systems at the Fukushima Dai’ichi nuclear power plant complex in Japan in March 2011, it triggered a sequence of events leading to one of the worst releases of radioactive materials in the world to date. Although nuclear energy is having a revival as a low-emissions energy source to mitigate climate change, the Fukushima accident is still cited as a reason for hesitancy in adopting it.
A new study synthesizes information from multidisciplinary sources to understand how the Fukushima Dai’ichi disaster unfolded, and points to the importance of mitigation measures and last lines of defense — even against accidents considered highly unlikely. These procedures have received relatively little attention, but they are critical in determining how severe the consequences of a reactor failure will be, the researchers say.
The researchers note that their synthesis is one of the few attempts to look at data across disciplinary boundaries, including: the physics and engineering of what took place within the plant’s systems, the plant operators’ actions throughout the emergency, actions by emergency responders, the meteorology of radionuclide releases and transport, and the environmental and health consequences documented since the event.
The study appears in the journal iScience , in an open-access paper by postdoc Ali Ayoub and Professor Haruko Wainwright at MIT, along with others in Switzerland, Japan, and New Mexico.
Since 2013, Wainwright has been leading the research to integrate all the radiation monitoring data in the Fukushima region into integrated maps. “I was staring at the contamination map for nearly 10 years, wondering what created the main plume extending in the northwest direction, but I could not find exact information,” Wainwright says. “Our study is unique because we started from the consequence, the contamination map, and tried to identify the key factors for the consequence. Other people study the Fukushima accident from the root cause, the tsunami.”
One thing they found was that while all the operating reactors, units 1, 2, and 3, suffered core meltdowns as a result of the failure of emergency cooling systems, units 1 and 3 — although they did experience hydrogen explosions — did not release as much radiation to the environment because their venting systems essentially worked to relieve pressure inside the containment vessels as intended. But the same system in unit 2 failed badly.
“People think that the hydrogen explosion or the core meltdown were the worst things, or the major driver of the radiological consequences of the accident,” Wainright says, “but our analysis found that’s not the case.” Much more significant in terms of the radiological release was the failure of the one venting mechanism.
“There is a pressure-release mechanism that goes through water where a lot of the radionuclides get filtered out,” she explains. That system was effective in units 1 and 3, filtering out more than 90 percent of the radioactive elements before the gas was vented. However, “in unit 2, that pressure release mechanism got stuck, and the operators could not manually open it.” A hydrogen explosion in unit 1 had damaged the pressure relief mechanism of unit 2. This led to a breach of the containment structure and direct, unfiltered venting to the atmosphere, which, according to the new study, was what produced the greatest amount of contamination from the whole weeks-long event.
Another factor was the timing of the attempt to vent the pressure buildup in the reactor. Guidelines at the time, and to this day in many reactors, specified that no venting should take place until the pressure inside the reactor containment vessel reached a specified threshold, with no regard to the wind directions at the time. In the case of Fukushima, an earlier venting could have dramatically reduced the impact: Much of the release happened when winds were blowing directly inland, but earlier the wind had been blowing offshore.
“That pressure-release mechanism has not been a major focus of the engineering community,” she says. While there is appropriate attention to measures that prevent a core meltdown in the first place, “this sort of last line of defense has not been the main focus and should get more attention.”
Wainwright says the study also underlines several successes in the management of the Fukushima accident. Many of the safety systems did work as they were designed. For example, even though the oldest reactor, unit 1, suffered the greatest internal damage, it released little radioactive material. Most people were able to evacuate from the 20-kilometer (12-mile) zone before the largest release happened. The mitigation measures were “somewhat successful,” Wainwright says. But there was tremendous confusion and anger during and after the accident because there were no preparations in place for such an event.
Much work has focused on ways to prevent the kind of accidents that happened at Fukushima — for example, in the U.S. reactor operators can deploy portable backup power supplies to maintain proper reactor cooling at any reactor site. But the ongoing situation at the Zaporizhzhia nuclear complex in Ukraine, where nuclear safety is challenged by acts of war, demonstrates that despite engineers’ and operators’ best efforts to prevent it, “the totally unexpected could still happen,” Wainwright says.
“The big-picture message is that we should have equal attention to both prevention and mitigation of accidents,” she says. “This is the essence of resilience, and it applies beyond nuclear power plants to all essential infrastructure of a functioning society, for example, the electric grid, the food and water supply, the transportation sector, etc.”
One thing the researchers recommend is that in designing evacuation protocols, planners should make more effort to learn from much more frequent disasters such as wildfires and hurricanes. “We think getting more interdisciplinary, transdisciplinary knowledge from other kinds of disasters would be essential,” she says. Most of the emergency response strategies presently in place, she says, were designed in the 1980s and ’90s, and need to be modernized. “Consequences can be mitigated. A nuclear accident does not have to be a catastrophe, as is often portrayed in popular culture,” Wainright says.
The research team included Giovanni Sansavini at ETH Zurich in Switzerland; Randall Gauntt at Sandia National Laboratories in New Mexico; and Kimiaki Saito at the Japan Atomic Energy Agency.
Reprinted with permission of MIT News